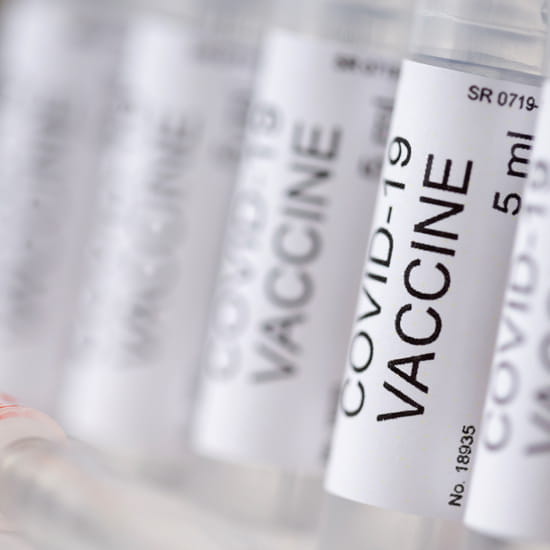
Published December 17, 2020
Moderna and Pfizer are implementing the first distribution of mRNA vaccines in the United States. Only recently have mRNA and plasmid DNA science gained traction as incredible tools for the rapid development of effective vaccines. Since this is the first time these types of agents will be used in the United States, primary care clinicians must understand how they work, the risks and benefits, and how they differ from other vaccines.
A Quick Refresher
In general, vaccines work by presenting a germ, or portion of it, to the body in order to teach the immune system what is foreign so it may fight the invader in the future. This process is called adaptive immunity, or immunity that develops as a person is exposed to pathogens. The adaptive immune system’s two major divisions are humoral immunity: B-cell antibody production, and cell-mediated immunity: T lymphocyte recognition of foreign, damaged cells. Cell-mediated immunity is further divided into helper (CD4+) and cytotoxic (CD8+) T cell responses. CD4+ cells help antibody production, while CD8+ cells help destroy damaged cells and clean up the mess through phagocytosis. After the invasion and fight, the immune response to a particular pathogen is incorporated into the system’s memory and protects future infection.
When the pathogen is reencountered, humoral immunity acts quickly to recognize foreign extracellular proteins. Cell-mediated immunity takes its time identifying intracellular viruses and microorganisms that need to be destroyed by cell lysis and phagocytosis. Historically, vaccines focus on creating humoral immunity or antibody production. This means that artificial immunity might not work as well as natural immunity since T cells are not widely involved. However, scientists have made discoveries that give T cells a more prominent role in artificial immunity.
- There are several types of vaccines available, and they include:
- Live attenuated vaccines
- Subunit, polysaccharide, recombinant protein, and conjugate vaccines
- Inactivated vaccines
- Toxoid vaccines
Recombinant viral vector vaccines are relatively new and are implemented in veterinary medicine. They hold promise for future human vaccination. The newest players on the market include plasmid DNA and mRNA vaccines. This article describes the differences between these vaccines. It explains why the new mRNA vaccines came to fruition quickly and are more highly effective than previously thought might be attainable.
How the mRNA Vaccine Works
The Moderna and Pfizer SARS-CoV-2 vaccines contain a piece of viral mRNA surrounded by a lipid coat. The snippet of mRNA is the code for the SARS-CoV-2 spike protein. The vaccine is injected into the upper arm. The lipid is taken up into muscle cells and dissolved, leaving the spike protein mRNA in the cell’s cytoplasm. At this point, the cell can use its own hardware to reverse transcribe the SARS-CoV-2 spike protein. After this process, cell enzymes dissolve the mRNA. The spike protein is displayed on the cell’s surface and soon is recognized as a foreigner, or antigen, by the immune system. After a few days of feeling mild viral symptoms related to the inflammatory response, not to SARS-CoV-2 itself, the body incorporates memory B and T cell knowledge into the immune system to fight another day. Scientists are not exactly sure how long the immunity will last, however.
Important Differences
No live virus, unlike live attenuated vaccines
- mRNA vaccines do not contain live viruses, so they do not risk causing disease in a vaccinated person. Live germs that are weakened through genetic manipulation for vaccination create a very similar immune response as that created by natural infection. However, weakened live germs can lead to infection in immunocompromised people, and they can be reactivated, causing actual infection in vaccinated individuals. These risks create much fear among the public. Therefore, the clinician needs to allay these fears by describing the true nature of the mRNA vaccine. Examples of live attenuated vaccines include measles, mumps, rubella, smallpox, chickenpox, and yellow fever.
Rapid production, unlike subunit, polysaccharide, recombinant protein, and conjugate vaccines
- The development of mRNA vaccines is standardized from a DNA template with readily available materials and quickly scaled up for fast production. Compared to other vaccines, like subunit, polysaccharide, recombinant protein, and conjugate vaccines, manufacturing times are significantly reduced. It takes a long time to develop subunit, polysaccharide, recombinant protein, and conjugate vaccines since they are human-made protein or sugar components. Examples of these vaccines include hepatitis B, pneumococcal disease, meningococcal disease, human papillomavirus, and Hemophilus influenzae.
Novavax and Sanofi, in partnership with GlaxoSmithKline, are other pharmaceutical companies working on protein-based SARS-CoV-2 vaccines, but production is taking longer.
Robust cytotoxic T cell response, unlike inactivated and toxoid vaccines
- To avoid the risks associated with live virus reactivation, scientists developed inactivated vaccines that use killed versions of a germ. In these vaccines, pathogens are inactivated by heat, chemicals, or radiation in a way that keeps key antigens intact for an appropriate immune response. Toxoid vaccines are similar, but the inactivated toxin produced by the pathogen is the antigenic agent that produces immunity. However, these types of vaccines, and the previously discussed subunit, polysaccharide, recombinant protein, and conjugate vaccines, do not create a robust immune response. This is in part because vaccine development typically relies on creating an excellent humoral immune response. This means that vaccines are good at producing antibodies, but they often do not elicit good T cell responses. In contrast to the older vaccine models, the Moderna and Pfizer research shows that the new mRNA vaccines produce powerful helper and cytotoxic T cell responses. Researchers think that full B and T cell responses contribute significantly to vaccine success.
- Older vaccines use multiple booster doses to overcome the weaker immune response, and the boosters work to provide immunity. The mRNA vaccines also require booster doses but for a different reason. Boosters are needed because mRNA is volatile and quickly broken down in cells. Examples of inactivated vaccines include hepatitis A, injection form influenza, injection form polio, and rabies. An example of a toxoid vaccine is tetanus.
mRNA Vaccines are Easily Denatured
- As a clinician might expect, mRNA is much less stable than DNA, and it is easily denatured. Previously, its transient nature made it less appealing as an avenue for vaccine development. However, scientists were able to create modified nucleosides that allow RNA to be stable and less immunogenic. This breakthrough recently advanced vaccine research. However, although more stable, mRNA vaccines still require freezing storage to remain intact. This is a big hurdle for Moderna and Pfizer since low-income countries have less ability to store these vaccines. The other SARS-CoV-2 vaccines under development do not require such cold storage.
Other Vaccine Options
Recombinant viral vector vaccines
- Two other SARS-CoV-2 vaccines under development use recombinant viral vector technology. AstraZeneca and Johnson and Johnson’s viral vector vaccines use a genetically altered minor adenovirus as a vector that holds SARS-CoV-2 genetic material. SARS-CoV-2 genetic material that codes for the spike protein is incorporated into the adenoviral genome before being introduced into the host. The cells take up the adenovirus, and an immune response is created against both the adenovirus and the SARS-CoV-2 proteins.
- To date, this type of vaccine is only used in veterinary medicine, but it holds great promise for success in humans. These vaccines can be highly effective since they produce good humoral and cell-mediated responses, but manufacturing can take a very long time. Additionally, if people have already had prior exposure to the chosen vector virus, the body could fight off the infection before adequately delivering and replicating the necessary SARS-CoV-2 genetic material. To combat this potential problem, the AstraZeneca vaccine uses a chimpanzee adenovirus that does not cause illness in humans.
Plasmid DNA vaccines
- Other new vaccine technology includes the use of plasmid DNA. None of the widely discussed SARS-CoV-2 vaccines are plasmid DNA vaccines, but this type of technology will appear soon. Another pharmaceutical company is beginning a clinical trial this month. A plasmid DNA vaccine is similar to an mRNA vaccine in that genetic material is given a lipid coat and then injected into human muscle cells. The DNA becomes incorporated into the host cell genome and codes for antigenic proteins that create an immune response. The most significant difference in mRNA vaccines is that the genetic material does not enter a cell’s nucleus to become part of a person’s genetic code.
Vaccine science has changed significantly over the last ten years, and new technologies hold substantial promise for helping us eradicate not only COVID-19, but malaria, HIV, and cancer.
For more insights, view our collection of COVID-19 resources and CME courses. We recognize it is critical that you have access to timely, reliable information, so we are working hard to release new content in collaboration with our team of infectious disease experts, medical specialists, and primary care clinicians. To hear more from these experts, sign up for a free virtual conference where you can earn CME/CE credit from the comfort of your home or office.
References
Centers for Disease Control. Understanding mRNA COVID-19 Vaccines. Accessed December 4, 2020. https://www.cdc.gov/coronavirus/2019-ncov/vaccines/different-vaccines/mrna.html.
Centers for Disease Control. Understanding and Explaining mRNA COVID-19 Vaccines. Accessed December 4, 2020. https://www.cdc.gov/vaccines/covid-19/hcp/mrna-vaccine-basics.html#:~:text=mRNA%20vaccines%20do%20not%20contain,interact%20with%20a%20person’s%20DNA.
National Institute of Allergy and Infectious Diseases. Vaccine Types. Updated July 1, 2019. Accessed Dec 4, 2020. https://www.niaid.nih.gov/research/vaccine-types.
U.S. Department of Health and Human Services. Vaccine Types. Accessed December 7, 2020. https://www.vaccines.gov/basics/types.
Xiaoxia Dai, Yongmin Xiong, Na Li, Can Jian. Vaccine Types, In: Vijay Kumar, ed. Vaccines – the History and Future. China: IntechOpen; March 5, 2019. Available from: https://www.intechopen.com/books/vaccines-the-history-and-future/vaccine-types.
Lumen Learning. Vaccines. In: OpenStax Microbiology. Accessed December 4, 2020. https://courses.lumenlearning.com/microbiology/chapter/vaccines/.
Liu MA. A Comparison of Plasmid DNA and mRNA as Vaccine Technologies. Vaccines (Basel). 2019;7(2):37. Published 2019 Apr 24. doi:10.3390/vaccines7020037
Gilbert SC. T-cell-inducing vaccines – what’s the future. Immunology. 2012;135(1):19-26. doi:10.1111/j.1365-2567.2011.03517.x
Lundstrom K. The Current Status of COVID-19 Vaccines. Frontiers in Genome Editing. 2020;2:1-10. https://www.frontiersin.org/articles/10.3389/fgeed.2020.579297/full.
Panogioti E, Klenerman P, Lee LN, van der Burg SH, Arens R. Features of Effective T Cell-Inducing Vaccines against Chronic Viral Infections. Frontiers in Immunology. 2018;9:1-11. https://www.frontiersin.org/articles/10.3389/fimmu.2018.00276/full.
The Vaccine Alliance. What are viral vector-based vaccines and how could they be used against COVID-19? Accessed Dec 4, 2020. https://www.gavi.org/vaccineswork/what-are-viral-vector-based-vaccines-and-how-could-they-be-used-against-covid-19.
Bendix A. Pfizer’s coronavirus vaccine relies on a new, unproven technology. A diagram shows how it differs from other candidates. Business Insider. Published Nov 9, 2020. Accessed Dec 4, 2020. https://www.businessinsider.com/leading-us-coronavirus-vaccines-how-they-work-compare-2020-10.
Johnson CY, Steckelberg A. What you need to know about the Pfizer, Moderna and AstraZeneca vaccines. The Washington Post. Updated Nov 30, 2020. Accessed Dec 7, 2020. https://www.washingtonpost.com/health/2020/11/17/covid-vaccines-what-you-need-to-know/?arc404=true.
Corum J, Zimmer C. How the Pfizer-BioNTech Vaccine Works. The New York Times. Updated Dec 7, 2020. Accessed Dec 7, 2020. https://www.nytimes.com/interactive/2020/health/pfizer-biontech-covid-19-vaccine.html.